Fusion: A Stellar Force Unleashed
Nuclear fusion, as the term implies, is the process where light atomic nuclei, such as deuterium and tritium, merge into heavier nuclei like helium, releasing vast energy in the process. This celestial dance of fusion, standing apart from chemical processes which transpire on an atomic or molecular scale, is not a realm marked by the conservation of matter; here, mass partially transforms into photons—pure energy—as accounted by Einstein’s mass-energy equivalence. It is this very mechanism that powers the vibrant or “main sequence” stars, including our Sun—a cosmic powerhouse of fusion energy.
How Does Fusion Occur?
In essence, nuclear fusion happens when two light, positively charged nuclei have sufficient energy to overcome their electrostatic repulsion and collide. Despite the repellent force, at high energies and direct collisions, they can come sufficiently close to each other to allow nuclear forces to bind them, resulting in fusion.
Cold Fusion vs. Hot Fusion
Among the variants of fusion, the most talked-about in scientific circles is hot fusion. Cold fusion, on the other hand—as intriguing as it is—remains speculative and beyond the scope of this exploration, primarily focused on the widely recognized force of hot fusion.
As with many forefront technologies like the internet, the development of artificial fusion technology also began with military attempts—the formidable hydrogen bomb, to be precise. To trace a simple timeline of hot fusion research:
- In 1920, Arthur Eddington proposed that hydrogen to helium fusion might be the primary source of stellar energy.
- By 1932, following Rutherford’s pioneering work, Mark Oliphant had achieved experimental fusion of hydrogen isotopes in the laboratory.
- In the 1930s, Hans Bethe outlined the principal fusion cycles of stars.
- In the early 1940s, as part of the Manhattan Project, nuclear fusion was researched for military ends. In 1951, fusion was achieved in a nuclear test.
- Then, on November 1, 1952, a large-scale fusion reaction was conducted during the Ivy Mike hydrogen bomb test.
Parallel to the journey from atomic bomb to civilian nuclear fission reactors, after the success of the hydrogen bomb test, controlled hot fusion research embarked for civil purposes from the 1950s.
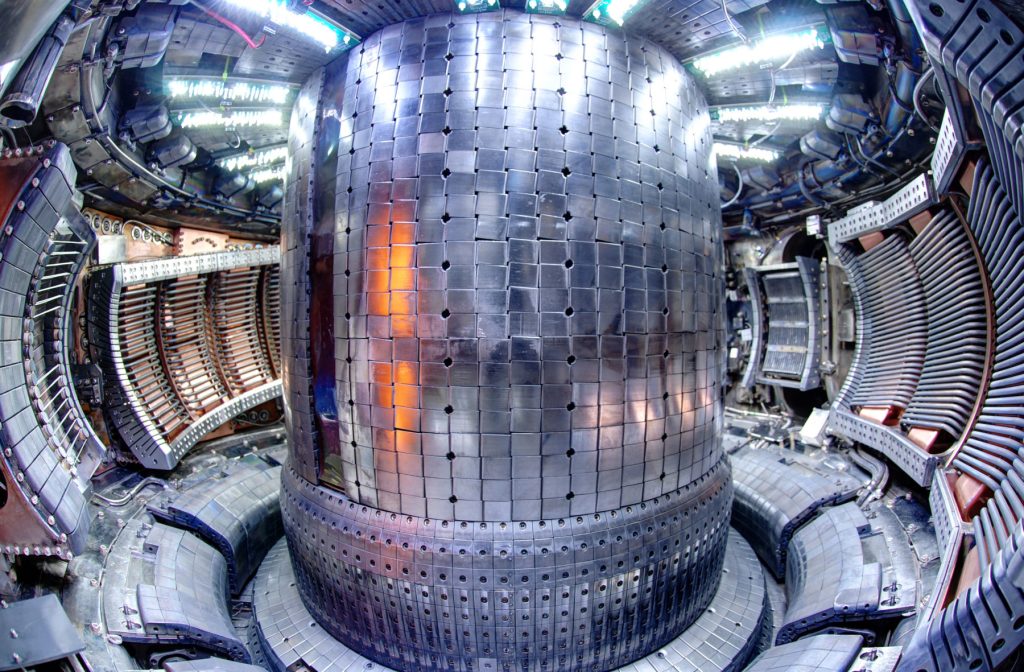
The Path to Controlled Fusion: Conditions and Research Trajectories
Humanity has managed uncontrolled fusion—like that seen in the explosion of a hydrogen bomb—and brief controlled fusion, albeit at an energy loss. For practical use, however, fusion must be rendered manageable, sustained, and stable in terms of energy output.
The Fusion Triple Product
Fusion first requires fuel in a plasma state—the fourth state of matter. Plasma—fully ionized and overall electrically neutral—is often called a ‘hot gas’. At high temperatures, electrons in a plasma have enough energy to escape atomic nuclei, setting the stage for fusion. If plasma reaches temperatures of tens to hundreds of millions of degrees with sufficient density and confinement time, fusion can sustain. The product of plasma’s temperature, density, and confinement time—the “triple product”—needs to reach a critical value for fusion to become self-sustaining.
Given the scale of the triple product, it’s no surprise that special containment is essential to achieve fusion. Currently, there are two main approaches: magnetic confinement fusion (MCF) and inertial confinement fusion (ICF).
ICF and MCF
ICF utilizes lasers or X-rays driving a spherical fuel pellet to create high-pressure plasma and ignite fusion, while MCF relies on magnetic fields to contain and heat plasma to fusion conditions. The leading edge of MCF research uses Tokamak technology.
Tokamaks and ITER
The word ‘tokamak’ comes from the Russian abbreviation of ‘toroidal chamber with magnetic coils’, encapsulating the design of this Soviet-devised reactor from the 1950s. Tokamaks have since proliferated and represent the frontier of fusion technology. Within its toroidal chamber, powerful magnets induce plasma currents and a confining spiral magnetic field, aspiring to sustain fusion.
Significant tokamak installations across the globe exemplify the strides made in MCF research. China’s EAST, or the ‘Eastern Superconducting Tokamak’, is one such cutting-edge facility contributing major milestones to the fusion cause.
As for ITER—the International Thermonuclear Experimental Reactor—in southern France, it is a collaboration launched in 1985, with the mission to demonstrate the feasibility of fusion as a power source without the negative byproducts. Not designed to produce electricity, ITER’s objectives are to achieve high fusion power output, lasting plasma states, and promote tritium breeding alongside other key technological advancements relevant to future fusion power plants.
Discussion about this post