Introduction: Overview of Quantum Computing and Its Potential
Quantum computing is often described as the next frontier in the field of technology, with the potential to revolutionize industries from cryptography and cybersecurity to pharmaceuticals and artificial intelligence. Unlike classical computers, which rely on bits to represent information as either 0 or 1, quantum computers use quantum bits, or qubits, which can exist in multiple states simultaneously thanks to the principles of quantum mechanics. This phenomenon, known as superposition, allows quantum computers to process vast amounts of information much more efficiently than traditional computers.
The potential applications of quantum computing are vast, and its capabilities could enable breakthroughs in areas that were previously thought to be insurmountable. From solving complex optimization problems to simulating molecular structures for drug discovery, quantum computing has the power to drive significant advancements across diverse sectors. However, despite the immense promise, quantum computing remains in the early stages of development, and the journey toward fully realized, scalable quantum computers is fraught with technical challenges.
This article will explore the fundamentals of quantum computing, its most promising applications, the current state of quantum research, and the challenges that need to be overcome for it to become a transformative force in technology.
How Quantum Computing Works: Basics of Quantum Mechanics in Computing
At the heart of quantum computing lies the fascinating world of quantum mechanics, the branch of physics that deals with the behavior of particles at the subatomic level. Unlike classical bits, which represent information as either a 0 or a 1, quantum bits or qubits can exist in a state of superposition. This means that a qubit can represent both 0 and 1 simultaneously, enabling quantum computers to perform many calculations in parallel.
Another key concept is entanglement. When qubits become entangled, the state of one qubit is directly related to the state of another, no matter how far apart they are. This interconnectedness allows quantum computers to solve certain problems exponentially faster than classical computers. When qubits are entangled, a change in one qubit will instantly affect the others, which provides a powerful means of computation.
Quantum interference is also critical to quantum computing. Just as waves can cancel each other out or reinforce one another, quantum states can interfere, amplifying the correct solutions and canceling out wrong ones. This interference allows quantum computers to navigate through multiple possibilities and select the optimal solution for certain complex problems.
Building a quantum computer requires creating and maintaining qubits in a controlled state. Because quantum states are fragile and easily disturbed by external factors, quantum computers must operate at extremely low temperatures, often just a few degrees above absolute zero. To date, researchers have been able to build small quantum processors, but scaling them to a level that can solve practical, real-world problems remains a significant challenge.
Applications in Cryptography: The Impact of Quantum Computing on Data Encryption and Cybersecurity
One of the most discussed applications of quantum computing is in the field of cryptography. Currently, encryption systems like RSA and ECC (Elliptic Curve Cryptography) rely on the difficulty of factoring large numbers or solving certain mathematical problems to secure data. These systems are considered secure because classical computers would take an impractically long time to crack these codes.
However, quantum computers pose a significant threat to these encryption methods. With quantum algorithms like Shor’s algorithm, a sufficiently powerful quantum computer could factor large numbers exponentially faster than any classical computer, breaking RSA and other encryption systems that rely on the hardness of these problems. This has profound implications for online security, banking, and personal privacy, as much of the world’s digital infrastructure relies on these encryption methods.
Quantum computers could also play a crucial role in developing new, quantum-resistant encryption algorithms. These quantum encryption techniques, such as Quantum Key Distribution (QKD), leverage the principles of quantum mechanics to create secure communication channels that are theoretically immune to eavesdropping. The ability to securely transmit information using the laws of quantum mechanics could revolutionize cybersecurity, particularly in areas such as financial transactions, national security, and private communications.
As quantum computing continues to evolve, the cybersecurity landscape will also need to adapt. The rise of quantum-resistant encryption algorithms and the integration of quantum key distribution systems into our communications infrastructure will be necessary to protect sensitive data from quantum-enabled threats.
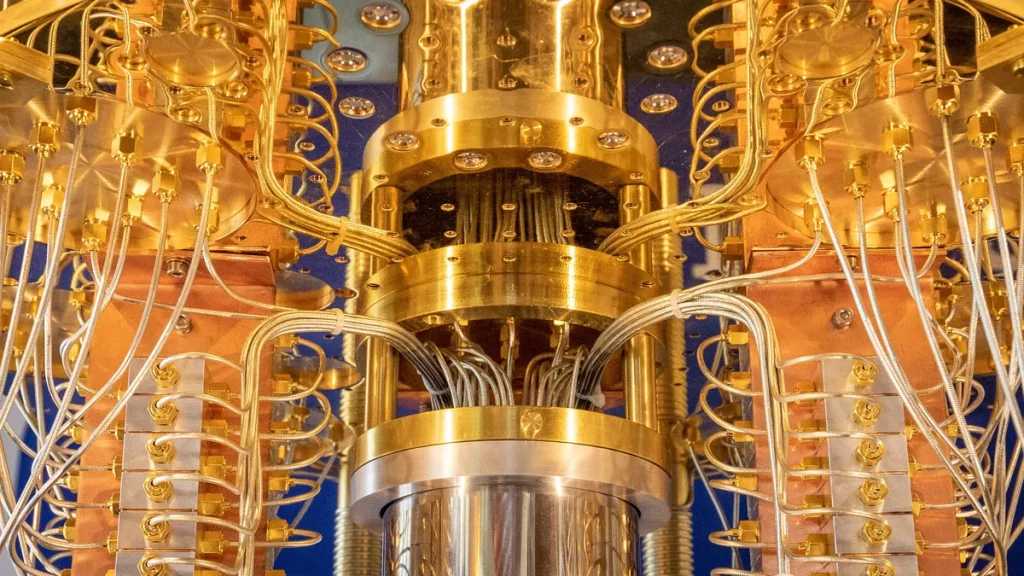
Quantum AI and Machine Learning: The Synergy Between Quantum Computing and Artificial Intelligence
Another exciting area where quantum computing is poised to make a significant impact is artificial intelligence (AI) and machine learning (ML). AI relies on processing vast amounts of data to recognize patterns, make predictions, and automate decision-making. However, as AI models become more complex, the computational demands required to train these models have grown exponentially. Quantum computing offers the potential to accelerate these processes, enabling AI and ML systems to learn from data more efficiently.
Quantum computing could speed up the training of machine learning models by using quantum superposition and entanglement to explore multiple solutions simultaneously. Quantum algorithms, like the quantum version of the well-known k-means clustering algorithm, could enable AI systems to find patterns in data much faster than classical computers, potentially opening up new possibilities for real-time data analysis and decision-making.
For example, quantum computing could significantly enhance optimization problems, a key area in machine learning. In tasks like logistics, scheduling, and resource allocation, quantum computers could process complex datasets with countless variables far more efficiently than classical computers, helping AI models to find the best possible solutions faster and with greater accuracy.
In addition, quantum computers could improve machine learning models in areas like quantum chemistry, where the complexity of simulating molecular structures makes classical computation infeasible. Quantum-enhanced AI could enable breakthroughs in fields such as drug discovery and material science by helping researchers simulate the behavior of molecules at a quantum level, something that is difficult or impossible for classical computers to achieve.
Challenges in Quantum Computing: Current Limitations and the Path to Scalable Quantum Computers
While the potential of quantum computing is immense, there are still significant hurdles to overcome before quantum computers can become a practical tool for solving real-world problems. One of the main challenges is decoherence—the loss of quantum information due to interactions with the external environment. Quantum states are extremely delicate, and qubits are prone to losing their quantum properties when exposed to noise, electromagnetic radiation, or other disturbances. Researchers are working on error correction techniques and better isolation methods, but decoherence remains one of the key obstacles to scaling quantum computers.
Another challenge is the creation of stable qubits. Current quantum computers rely on qubits made from materials like superconducting circuits or trapped ions, both of which have limitations in terms of stability and scalability. These systems need to be operated at extremely low temperatures, which requires specialized equipment and makes the process expensive and energy-intensive.
Quantum error correction is another area that needs significant development. Quantum computers are highly sensitive to errors due to the fragile nature of quantum states. Unlike classical computers, where errors can be easily detected and corrected, quantum computers require complex error-correction protocols that involve using additional qubits to detect and correct errors without disrupting the computation. Developing effective error correction schemes that do not require a massive overhead in terms of qubit resources is a critical challenge for building large-scale quantum computers.
Finally, there is the issue of scalability. Building a quantum computer with enough qubits to outperform classical computers on practical tasks requires a massive increase in the number of qubits and the ability to manipulate them with precision. Researchers are exploring various approaches to scaling quantum computers, but as of now, quantum processors with hundreds or thousands of qubits remain elusive.
Conclusion: The Transformative Potential of Quantum Computing in Various Industries
Quantum computing holds transformative potential for many industries, including healthcare, finance, logistics, and artificial intelligence. From enhancing cybersecurity to optimizing complex systems and enabling breakthroughs in drug discovery, quantum computing could address challenges that were once thought to be unsolvable by classical computers.
However, as we look to the future of quantum computing, it is important to recognize that we are still in the early stages of this technological revolution. While quantum computers have demonstrated impressive results in laboratory settings, they have not yet reached the scale and reliability required for real-world applications. The road to practical, large-scale quantum computers will require overcoming significant technical hurdles, including issues of stability, error correction, and scalability.
As researchers continue to push the boundaries of what is possible with quantum computing, industries around the world must prepare for a future where quantum technology plays an integral role in shaping the next generation of computing. The promise of quantum computing is immense, and with ongoing advances in research and development, it may soon become a cornerstone of our digital future.
Discussion about this post