Fermionic Foundations in Quantum Mechanics
In the realm of physics, protons, neutrons, and electrons are unanimously classified as fermions, fundamentally differentiated by their intrinsic spin—a half-integer multiple of Planck’s constant divided by $2\pi, manifesting as values like \1/2, \3/2, \5/2$, and so on.
The Pauli Exclusion Principle
Guided by the spin-statistics theorem, fermions adhere to the Pauli Exclusion Principle. This quintessential quantum protocol asserts that no two fermions in a system can occupy the same quantum state simultaneously. This principle not only lays bare the structure of atomic shells and the tableau of elements on the periodic table, it reinforces the stability of the material universe we inhabit.
Predictions and Pursuits in the Fermi Sea
Three decades ago, physicists envisioned a scenario linked deeply to the essence of fermions. They postulated that in a cold gas comprised of fermions, the scattering of light is suppressed, a phenomenon aptly termed as Pauli blocking. Elusive and subtlety defined, observing Pauli Blocking requires extreme experimental conditions—high particle densities paired with cryogenic temperatures.
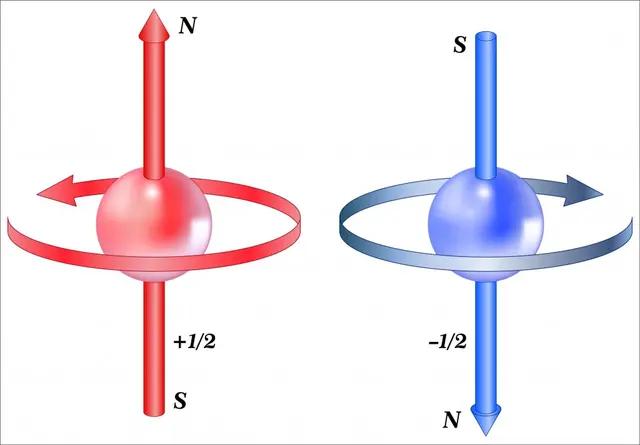
Empirical Glimpses of Quantum Suppression
Now, the prestigious journal ‘Science’ heralds the publication of three independent research papers. These detailed reports herald the inaugural experimental verification of Pauli blocking, the enigmatic phenomenon long-predicted but never observed, until now.
In the paradigmal scenario, photons navigate an atomic cloud, colliding and scattering much like billiard balls, rendering the atomic agglomeration visible. Yet, Pauli blocking forecasts that when atoms are chilled and compressed, their effective scattering space dwindles; photons traverse unscattered, as if the atoms slip into transparency.
An Analogy in an Arena
Consider the analogy of seating within a sports stadium for Pauli blocking. Each patron—an atom—each seat—a quantum state. An atom must migrate to a vacant seat to absorb a photon’s impact to scatter it. Should all adjacent seats be occupied, the atom loses the capacity to absorb and scatter, effectively becoming transparent.
Overcoming Density Barriers
Until recently, achieving sufficient density was a significant hurdle, with less dense scenarios allowing atoms “seats” to scatter light. Modern physicists globally have advanced magnetic and laser-based techniques to reach ultracold conditions.
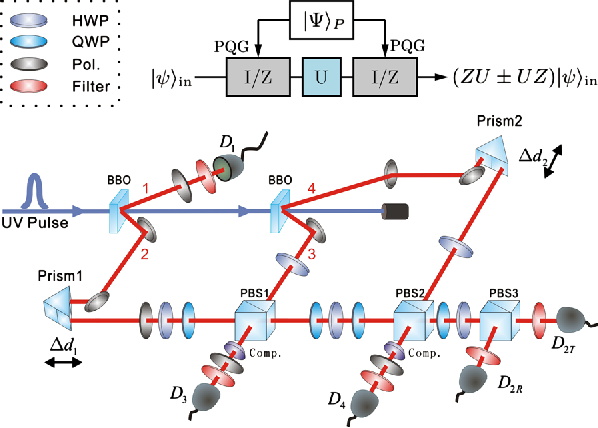
Triumph of Experimental Collaboration
Teams from the National Institute of Standards and Technology (NIST) in the United States, the University of Otago in New Zealand, and the Massachusetts Institute of Technology (MIT) have verified this fundamental and peculiar quantum phenomenon by magnetically trapping atoms and cooling them near absolute zero.
These groups, each employing distinct atomic species—strontium, potassium, and lithium, respectively—have tailored dense atomic gases into crowded ‘fermi seas’. Despite their divergent experimental approaches, they share a core feature: reducing the atomic gas’ energy to the purest quantum mechanical limits. Their collective findings are strikingly consistent: as gas temperatures dip and densities climb to craft a fermi sea, light scattering by the gas perceptibly diminishes.
Taking NIST’s experiment as an exemplar, researchers excited the fermi sea’s strontium atoms with blue light, subsequently measuring the photons radiating in assorted directions. They noted a 50% reduction in photon scattering at narrow angles. At MIT, when lithium atoms were cooled to a mere 20 microkelvin, a 38% decrease in atomic brightness ensued.
The Quantum Effect with Far-Reaching Implications
Pauli blocking is a profound quantum effect with potential to manipulate heretofore immutable properties of matter. Prior to this new wave of research, theorists had posited the embedding of atoms into a fermi sea to study Pauli blocking—now, researchers have transformed this from supposition to actuality. This method offers fresh techniques for quantum engineering of atomic light systems, with budding applications. By affirming that Pauli Blocking can indeed impact an atom’s capacity to scatter light, scientists might better craft materials that suppress light scattering, potentially preserving data in quantum computers.
Discussion about this post