Introduction
In a landmark achievement for space science and quantum technology, NASA has successfully demonstrated an ultra-cool quantum sensor aboard the International Space Station (ISS). This groundbreaking experiment represents a significant leap forward in our ability to measure and understand fundamental physical phenomena in the cosmos. The demonstration not only highlights NASA’s commitment to advancing scientific knowledge but also showcases the potential of quantum sensors in revolutionizing space exploration and fundamental research.
Quantum sensors operate at temperatures close to absolute zero, where quantum effects dominate and classical physics no longer applies. By harnessing these quantum effects, scientists can achieve unprecedented levels of precision and sensitivity. This technology has the potential to transform our understanding of the universe, from detecting gravitational waves to probing the mysteries of dark matter and dark energy.
In this article, we will explore the intricacies of NASA’s ultra-cool quantum sensor demonstration, delve into the principles of quantum sensing, discuss the significance of the experiment aboard the ISS, and consider the future implications for space science and technology.
Quantum Sensing: Principles and Applications
Understanding Quantum Sensors
Quantum sensors leverage the principles of quantum mechanics to measure physical quantities with extreme precision. Unlike classical sensors, which rely on macroscopic measurements, quantum sensors exploit quantum states and their interactions to achieve unparalleled sensitivity. These sensors typically operate at temperatures close to absolute zero, where quantum effects such as superposition and entanglement become significant.
Key Principles:
- Superposition: Quantum superposition allows particles to exist in multiple states simultaneously. This property enables quantum sensors to explore a range of possible states, leading to highly sensitive measurements.
- Entanglement: Quantum entanglement is a phenomenon where particles become interconnected in such a way that the state of one particle instantly affects the state of another, regardless of distance. Entangled particles can be used to enhance measurement precision and accuracy.
- Quantum Interference: Quantum interference occurs when quantum states overlap, creating interference patterns that can be used to measure physical quantities with high precision.
Types of Quantum Sensors
Quantum sensors come in various forms, each designed for specific applications. Some of the most notable types include:
- Atomic Clocks: These sensors measure time with incredible accuracy by counting the vibrations of atoms. Atomic clocks are crucial for GPS systems, telecommunications, and fundamental physics research.
- Gravitational Wave Detectors: Instruments like LIGO use quantum sensors to detect gravitational waves—ripples in spacetime caused by massive cosmic events. These detectors are sensitive to minute changes in distance caused by passing gravitational waves.
- Magnetometers: Quantum magnetometers measure magnetic fields with high precision. They are used in geological surveys, medical imaging, and fundamental research in physics.
- Interferometers: Quantum interferometers measure small displacements or changes in optical paths using interference patterns. They are employed in various scientific experiments, including studies of gravitational waves and fundamental constants.
The Ultra-Cool Quantum Sensor Experiment
The Cold Atom Laboratory (CAL)
NASA’s Cold Atom Laboratory (CAL) on the ISS is a state-of-the-art facility designed to study ultra-cold quantum gases and conduct precision measurements in a microgravity environment. CAL provides a unique platform for exploring quantum phenomena in conditions that are not achievable on Earth.
Key Features of CAL:
- Microgravity Environment: The ISS’s microgravity environment allows scientists to create and study ultra-cold atomic gases with unprecedented precision. The lack of gravity-related disturbances enables more accurate measurements of quantum effects.
- Advanced Cooling Techniques: CAL employs advanced laser cooling and evaporative cooling techniques to achieve temperatures close to absolute zero. These techniques are crucial for creating and maintaining ultra-cold atomic samples.
- Precision Measurement Tools: CAL is equipped with high-precision measurement tools, including laser systems and optical lattices, to study the behavior of ultra-cold atoms and perform quantum experiments.
The Quantum Sensor Demonstration
The ultra-cool quantum sensor demonstrated by NASA is based on a technology known as a quantum-enhanced accelerometer. This sensor measures accelerations with exceptional accuracy by exploiting the properties of ultra-cold atoms trapped in an optical lattice.
Demonstration Details:
- Sensor Operation: The quantum-enhanced accelerometer operates by measuring the interference patterns of ultra-cold atoms in an optical lattice. The sensor is highly sensitive to changes in acceleration, making it suitable for detecting tiny variations in gravitational fields and other accelerative forces.
- Experimental Setup: The experiment involves cooling atoms to near absolute zero, trapping them in a lattice created by laser beams, and then measuring their quantum interference patterns as they are subjected to different accelerative forces.
- Results and Observations: The demonstration successfully showed that the quantum-enhanced accelerometer could measure accelerations with unprecedented precision. The data obtained from the experiment will contribute to a better understanding of fundamental physical phenomena and the development of advanced sensing technologies.
Significance of the Experiment Aboard the ISS
Advancing Scientific Knowledge
The ultra-cool quantum sensor experiment aboard the ISS has several important implications for scientific research:
- Precision Measurements: The ability to measure accelerations with high precision opens new avenues for exploring fundamental physical constants and testing theories of physics. The data obtained from the experiment will help refine our understanding of gravity and other fundamental forces.
- Studying Quantum Phenomena: The ISS’s microgravity environment allows scientists to study quantum phenomena in conditions that are not achievable on Earth. This research can provide insights into the behavior of ultra-cold atoms and the nature of quantum effects.
- Technological Advancements: The development of quantum-enhanced accelerometers and other quantum sensors has the potential to revolutionize various fields, including navigation, space exploration, and fundamental physics research. The technology demonstrated in this experiment could lead to new applications and improvements in sensor technology.
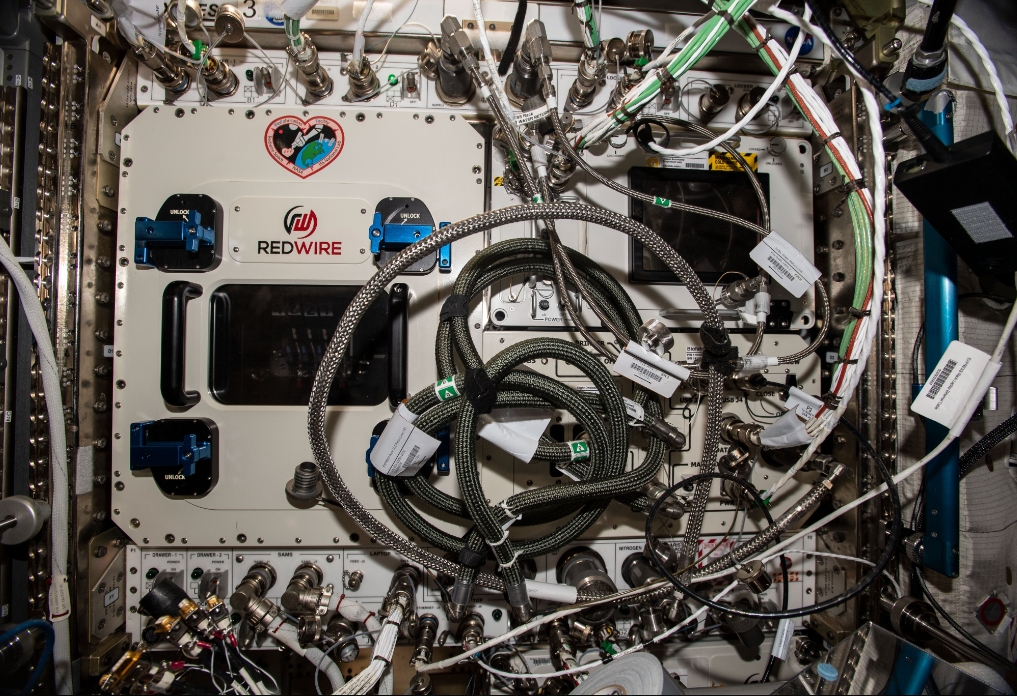
Implications for Space Exploration
The successful demonstration of the ultra-cool quantum sensor has several implications for space exploration:
- Enhanced Space Navigation: Quantum-enhanced sensors can provide highly accurate measurements of spacecraft acceleration and position. This capability could improve navigation systems for deep space missions, enabling more precise trajectory adjustments and maneuvering.
- Gravitational Research: Understanding gravitational fields with high precision is crucial for studying celestial bodies and phenomena. The data from the quantum sensor experiment could contribute to our understanding of gravitational fields around planets, moons, and other celestial objects.
- Fundamental Physics Research: The insights gained from the experiment could lead to new discoveries in fundamental physics, including the nature of gravity, quantum mechanics, and the fundamental forces of the universe.
Broader Impacts on Technology and Society
The advancements in quantum sensing technology demonstrated by NASA have broader impacts beyond space exploration:
- Medical Applications: Quantum sensors have potential applications in medical imaging and diagnostics. High-precision magnetometers and interferometers could improve imaging techniques and enhance our understanding of biological systems.
- Industrial Applications: Quantum sensors can be used in various industrial applications, including materials testing, environmental monitoring, and resource exploration. The technology could lead to improvements in manufacturing processes and resource management.
- Scientific Research: The development of advanced quantum sensors will benefit a wide range of scientific research fields, including materials science, chemistry, and fundamental physics. The technology will enable researchers to explore new phenomena and gain deeper insights into complex systems.
Future Directions and Research Opportunities
Advancements in Quantum Technology
The successful demonstration of the ultra-cool quantum sensor marks a significant milestone in quantum technology. Future research will focus on advancing quantum sensors and exploring new applications:
- Improving Sensor Precision: Researchers will continue to refine quantum sensors to achieve even higher levels of precision and sensitivity. Advances in cooling techniques, atom trapping, and measurement methods will contribute to the development of next-generation sensors.
- Exploring New Quantum Phenomena: The study of ultra-cold atoms and quantum effects will lead to new discoveries and insights into the fundamental nature of the universe. Researchers will explore new quantum phenomena and investigate their implications for physics and technology.
- Developing Practical Applications: The technology demonstrated in the experiment has the potential to be applied in various practical contexts. Researchers will explore applications in navigation, medical diagnostics, environmental monitoring, and other fields.
Collaboration and International Partnerships
The success of the quantum sensor experiment highlights the importance of collaboration and international partnerships in advancing space science and technology:
- Global Collaboration: International collaborations between space agencies, research institutions, and technology companies will drive advancements in quantum sensing and related fields. Collaborative efforts will enable the sharing of knowledge, resources, and expertise.
- Public-Private Partnerships: Partnerships between public space agencies and private industry will foster innovation and accelerate the development of new technologies. Public-private collaborations will contribute to the advancement of quantum sensors and their applications.
Long-Term Vision for Space Exploration
NASA’s demonstration of the ultra-cool quantum sensor is part of a broader vision for the future of space exploration:
- Exploring the Cosmos: The advancements in quantum sensing technology will support NASA’s long-term goals of exploring the cosmos and understanding the fundamental nature of the universe. Future missions will leverage these technologies to investigate distant celestial bodies and phenomena.
- Enhancing Human Spaceflight: Quantum sensors will play a role in enhancing human spaceflight missions, improving navigation, and ensuring the safety and success of deep space exploration.
- Pioneering New Frontiers: The development of advanced sensing technologies will enable humanity to pioneer new frontiers in space exploration, from studying the outer reaches of the solar system to investigating the mysteries of dark matter and dark energy.
Conclusion
NASA’s demonstration of the ultra-cool quantum sensor aboard the ISS represents a landmark achievement in the field of quantum technology and space science. By harnessing the principles of quantum mechanics and leveraging the unique conditions of the ISS
Discussion about this post